Modeling of electron transfer in DNA
The elucidation of the mechanisms of charge transfer in biomacromolecules is currently one of the most important problems of molecular biophysics and biochemistry, which is dealt with in a number of experimental works.
Now it is generally recognized that a DNA molecule in equilibrium state does not have any free charge carriers. Excess electrons (anion-radicals) or holes (cation-radicals) can be introduced into a DNA either due to photoexcitation upon exposure of the molecule to ultraviolet radiation or as a result of chemical reactions, for example, intercalation of some special molecular complexes. A possibility of proton transfer in a DNA is also considered. However, of specific interest is the study of the transfer of electrons and holes along a chain of base pairs, since the motion of radicals along a DNA molecule resulting in its destruction may possibly play a crucial role in the processes of mutagenesis and carcinogenesis. Experimental studies of charge transfer over DNA fragments have become practical only recently due to, on the one hand, progress in nano- and femtosecond technology, and on the other hand, development of biochemical methods of covalent binding of molecular complexes (which play the role of a donor and an acceptor) to a DNA fragment with already known sequence. In most experiments electrons or holes have been created in a special way in DNA fragments with known sequence of base pairs. The electron transport rate in this case has been calculated either from measurements of fluorescence quenching or from the analysis of the relative extent of damage produced in various sites of a DNA helix during the process of charge transfer. During the past few years huge experimental work has been done to elucidate the mechanism of charge transfer in DNA, however the problem is still to be solved.
Experiments have demonstrated that electrons and holes obtained by ionization method can spread over a region containing a number of base pairs. At room temperature such charge carriers can move along a DNA chain over considerable distances.
Even the first experiments have demonstrated a wide scatter in the values of the transfer rate (which differed by more than six orders of magnitude) as well as the dependence of this rate on such parameters as the type of a nucleotide sequence, the cause of excitations of an electron or a hole, the type of molecular complexes which serve as donors and acceptors of an electron, and the way of binding of such complexes to a DNA.
Despite considerable experimental evidence on charge transfer in DNA, theoretical works on the problem are still few in number and cannot explain the whole body of experimental data. Our laboratory has accumulated great experience in the study of electron transfer in proteins. We undertook these investigations in the framework of the RFBR project “Modeling of electron extended states in proteins and clusters” (project number 95-04-11432) and continued the work within the project “Long-range electron transfer in proteins and clusters via electron extended states” (project number 98-04-48828). While working at these problems we have developed a theory of electron states and long-range electron transfer in self-exchange reactions in globular proteins. The results obtained have enabled us to interpret the available experimental data on long-range electron transfer. The theory developed has provided a basis for modeling long-range electron transfer in DNA fragments containing tens of base pairs. In our approach we relied on a superexchange mechanism of electron transfer in DNA via electron extended states. The rate of electron transfer between rhodium-ruthenium complexes intercalated into a DNA has been calculated ( /2/, /3/ ). With the help of supercomputer computations we have studied quantum-mechanical evolution of a hole on a short DNA fragment in an irreversible reaction of a hole transfer between guanine and guanine triplet separated by a thymine dimer ( /1/, /4/, /5/ ). We believe that the experience accumulated by our laboratory in modeling the dynamics of electron transfer over short DNA fragments will be extended to the sequences containing hundreds of nucleotide pairs.
The work on the subject has been supported by the RFBR (project №01-07-90317).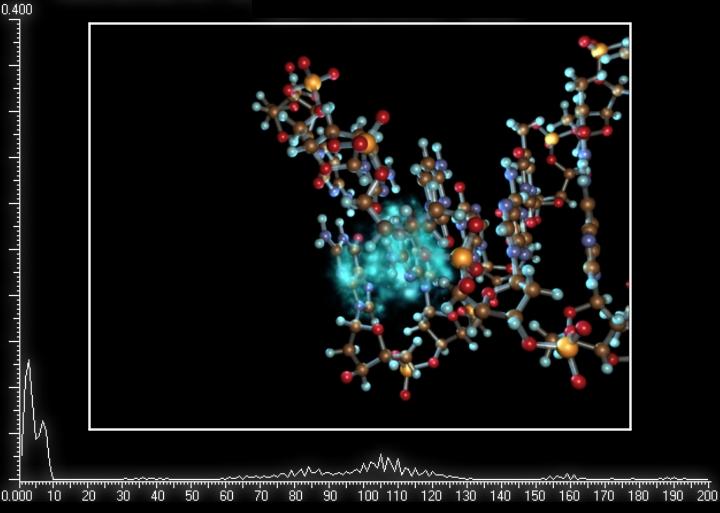
Now it is generally recognized that a DNA molecule in equilibrium state does not have any free charge carriers. Excess electrons (anion-radicals) or holes (cation-radicals) can be introduced into a DNA either due to photoexcitation upon exposure of the molecule to ultraviolet radiation or as a result of chemical reactions, for example, intercalation of some special molecular complexes. A possibility of proton transfer in a DNA is also considered. However, of specific interest is the study of the transfer of electrons and holes along a chain of base pairs, since the motion of radicals along a DNA molecule resulting in its destruction may possibly play a crucial role in the processes of mutagenesis and carcinogenesis. Experimental studies of charge transfer over DNA fragments have become practical only recently due to, on the one hand, progress in nano- and femtosecond technology, and on the other hand, development of biochemical methods of covalent binding of molecular complexes (which play the role of a donor and an acceptor) to a DNA fragment with already known sequence. In most experiments electrons or holes have been created in a special way in DNA fragments with known sequence of base pairs. The electron transport rate in this case has been calculated either from measurements of fluorescence quenching or from the analysis of the relative extent of damage produced in various sites of a DNA helix during the process of charge transfer. During the past few years huge experimental work has been done to elucidate the mechanism of charge transfer in DNA, however the problem is still to be solved.
Experiments have demonstrated that electrons and holes obtained by ionization method can spread over a region containing a number of base pairs. At room temperature such charge carriers can move along a DNA chain over considerable distances.
Even the first experiments have demonstrated a wide scatter in the values of the transfer rate (which differed by more than six orders of magnitude) as well as the dependence of this rate on such parameters as the type of a nucleotide sequence, the cause of excitations of an electron or a hole, the type of molecular complexes which serve as donors and acceptors of an electron, and the way of binding of such complexes to a DNA.
Despite considerable experimental evidence on charge transfer in DNA, theoretical works on the problem are still few in number and cannot explain the whole body of experimental data. Our laboratory has accumulated great experience in the study of electron transfer in proteins. We undertook these investigations in the framework of the RFBR project “Modeling of electron extended states in proteins and clusters” (project number 95-04-11432) and continued the work within the project “Long-range electron transfer in proteins and clusters via electron extended states” (project number 98-04-48828). While working at these problems we have developed a theory of electron states and long-range electron transfer in self-exchange reactions in globular proteins. The results obtained have enabled us to interpret the available experimental data on long-range electron transfer. The theory developed has provided a basis for modeling long-range electron transfer in DNA fragments containing tens of base pairs. In our approach we relied on a superexchange mechanism of electron transfer in DNA via electron extended states. The rate of electron transfer between rhodium-ruthenium complexes intercalated into a DNA has been calculated ( /2/, /3/ ). With the help of supercomputer computations we have studied quantum-mechanical evolution of a hole on a short DNA fragment in an irreversible reaction of a hole transfer between guanine and guanine triplet separated by a thymine dimer ( /1/, /4/, /5/ ). We believe that the experience accumulated by our laboratory in modeling the dynamics of electron transfer over short DNA fragments will be extended to the sequences containing hundreds of nucleotide pairs.
The work on the subject has been supported by the RFBR (project №01-07-90317).
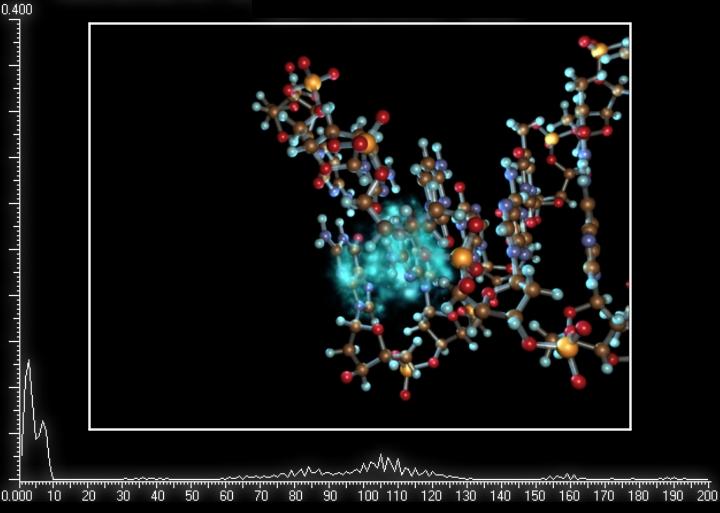